Nuclear Thermal Propulsion: The Key to Faster and More Efficient Deep Space Travel?
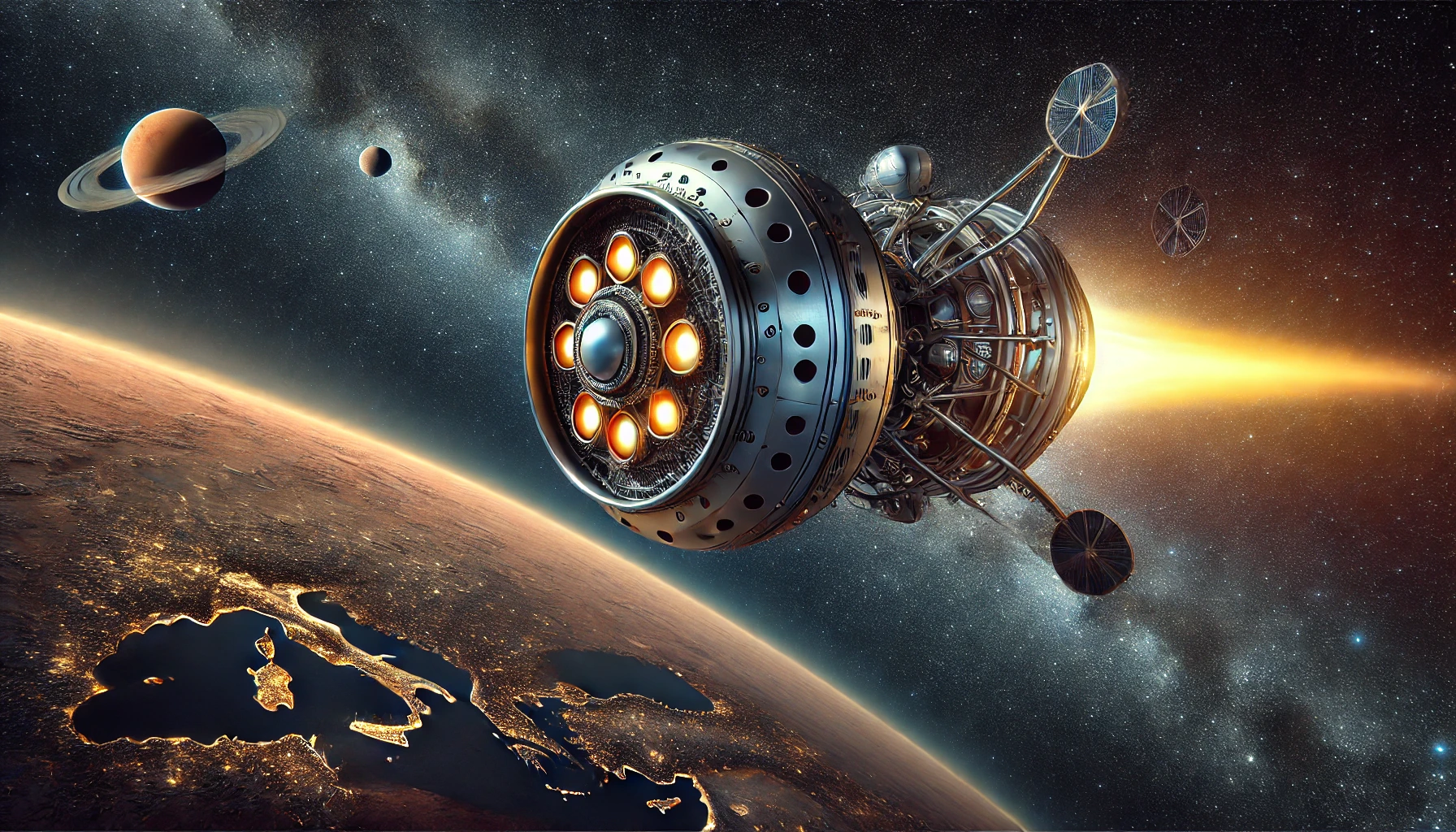
Introduction
Ever felt that interplanetary travel is a bit like watching paint dry? If you think waiting for your pizza delivery is agonizing, imagine astronauts spending months cooped up en route to Mars. As humanity sets its sights on the Red Planet and beyond, the glaring issue isn't just getting there—it's getting there before our favorite Netflix series gets another season. Enter nuclear thermal propulsion (NTP), the cosmic equivalent of swapping your bicycle for a rocket-powered skateboard. This technology promises to cut travel time to Mars and beyond, making deep space exploration not just a dream, but a more efficient reality. But what exactly is NTP, and why is it generating so much buzz in the space community? Let's dive into the nuclear-powered rabbit hole and find out.
The Limitations of Current Propulsion Methods
Traditional chemical rockets are like the reliable family sedan—dependable but not exactly thrilling in the speed department. These rockets rely on the combustion of chemical propellants to produce thrust, and while they've served us well since the days of Sputnik and Apollo, they're hitting a performance ceiling that's hard to ignore. For instance, a mission to Mars using conventional propulsion takes about nine months, according to NASA's mission profiles. That's nine months of astronauts floating in microgravity, battling muscle atrophy, exposure to cosmic radiation, and perhaps worst of all, the limited menu options of space cuisine. Moreover, the efficiency of chemical rockets is limited by the energy density of chemical bonds. The specific impulse (Isp), a measure of propulsion efficiency, for chemical rockets tops out around 450 seconds. This limitation not only affects travel time but also the amount of payload that can be transported. More fuel means less room for scientific instruments, habitats, or that extra pair of astronaut socks. The limitations aren't just about speed and payload. Long-duration missions pose significant risks to astronaut health. Extended exposure to cosmic radiation increases the risk of cancer and other health issues. According to a study published in the Journal of Space Radiation Research in 2022, astronauts on a round-trip mission to Mars could be exposed to radiation doses exceeding NASA's career limits. Reducing travel time isn't just a convenience—it's a necessity for the safety and well-being of the crew. In summary, while chemical propulsion has been the backbone of space exploration, it's increasingly evident that to venture further and faster, we need a new propulsion paradigm. And that's where nuclear thermal propulsion enters the scene, promising to address many of these challenges with a hefty dose of atomic efficiency.
Nuclear Thermal Propulsion Explained
So, what's the big deal with nuclear thermal propulsion? In simple terms, NTP uses a nuclear reactor to heat a propellant like liquid hydrogen. The reactor's core reaches temperatures upwards of 2,700 Kelvin, turning the liquid hydrogen into an extremely hot gas. This gas expands rapidly and is expelled out of the rocket nozzle at high velocities, producing thrust. It's like replacing your car's engine with a jet turbine—only way cooler and, you know, nuclear. The efficiency of a propulsion system is often measured by its specific impulse. NTP engines can achieve an Isp of around 900 seconds, roughly double that of the best chemical rockets. This means spacecraft can travel further on less fuel, or get to the same destination in less time. According to the American Nuclear Society, using NTP could reduce the transit time to Mars from nine months to as little as four to six months. That’s shaving off nearly half the time astronauts would be exposed to the perils of deep space. But let's not gloss over the 'nuclear' part. The reactor at the heart of an NTP system is similar to those used in nuclear submarines but designed to operate at much higher temperatures. Materials science plays a critical role here; the reactor components must withstand extreme temperatures and radiation. Advances in high-temperature ceramics and alloys are making these reactors more feasible. Additionally, modern reactor designs incorporate numerous safety features, such as control rods and fail-safe mechanisms, to prevent meltdowns or unintended reactions. Another advantage of NTP is the potential for higher thrust-to-weight ratios compared to other advanced propulsion concepts like electric or ion drives. While electric propulsion is highly efficient, it provides low thrust, making it unsuitable for crewed missions where time is of the essence. NTP strikes a balance between efficiency and thrust, offering a practical solution for near-term deep space missions. In essence, nuclear thermal propulsion isn't just about going faster—it's about enabling missions that are currently beyond our reach due to the limitations of existing technology. It opens the door to more ambitious exploration, from manned missions to Mars to robotic probes venturing to the outer planets and their moons.
Recent Advancements and Startups Leading the Way
In the past few years, a new wave of space startups has been shaking up the industry, bringing fresh perspectives and innovative solutions to long-standing challenges. Companies like Ultra Safe Nuclear Technologies (USNC-Tech) and X-energy are at the forefront of developing modular nuclear reactors designed specifically for space applications. USNC-Tech, for instance, unveiled a concept for a nuclear thermal propulsion engine in 2020 that leverages low-enriched uranium fuel, which addresses some of the proliferation and safety concerns associated with highly enriched uranium. In 2021, DARPA announced the Demonstration Rocket for Agile Cislunar Operations (DRACO) program, partnering with industry leaders like General Atomics and Blue Origin to develop and demonstrate an NTP system by 2025. This ambitious timeline reflects the urgency and confidence in NTP technology. According to a DARPA press release, the DRACO program aims to enable 'rapid maneuver in the space domain,' which could have significant implications not just for exploration but also for national security. NASA isn't sitting on the sidelines either. The agency's Nuclear Thermal Propulsion project is exploring reactor designs and materials, with plans to conduct ground-based tests in the near future. In 2022, NASA awarded contracts totaling $15 million to three companies—BWX Technologies, General Atomics, and Ultra Safe Nuclear Technologies—to develop reactor design concepts. These investments signify a renewed interest and commitment to making NTP a reality. Startups aren't just focusing on the reactors themselves. Companies like NeutronStar Systems are working on advanced materials and cooling systems critical for NTP. Their innovations aim to improve reactor efficiency and lifespan, addressing one of the key technical hurdles in NTP development. Furthermore, international collaboration is playing a role. The UK Space Agency, in partnership with Rolls-Royce, announced in 2021 a study into nuclear propulsion for deep space missions. Their research focuses on reactor designs that could be integrated into spacecraft, potentially accelerating the timeline for operational NTP systems. These collective efforts represent a significant shift from theoretical research to practical development. With multiple entities investing resources and talent, the race to achieve functional nuclear thermal propulsion is heating up—pun absolutely intended.
Challenges and Safety Concerns
Of course, introducing the word 'nuclear' into anything tends to raise eyebrows faster than a plot twist in a sci-fi movie. Safety is a significant concern, both in terms of operational safety and environmental impact. One of the primary risks is the potential release of radioactive material during a launch failure. Rockets, unfortunately, do have a tendency to explode occasionally—a fact not lost on regulators or the public. To mitigate this, engineers are designing reactors that remain inactive until the spacecraft reaches a safe orbit. This 'cold start' approach ensures that in the event of a launch failure, no nuclear reactions would have occurred, and the radioactive material would be contained within robust shielding. According to a 2023 report by the International Atomic Energy Agency (IAEA), advancements in fuel fabrication and containment could reduce the risk of radioactive exposure by up to 90% compared to earlier designs. Another challenge is managing the reactor's heat in the vacuum of space. Space isn't entirely cold—it's just really good at not conducting heat. Without an atmosphere, getting rid of excess heat is tricky. Engineers are exploring radiative cooling systems and heat pipe technologies to effectively dissipate heat from the reactor, ensuring it doesn't overheat. Regulatory hurdles are another mountain to climb. Launching nuclear material into space requires navigating a complex web of international treaties and national regulations. The United Nations Office for Outer Space Affairs (UNOOSA) has guidelines that must be followed, and individual countries have their own rules and approval processes. This can lead to lengthy delays and significant legal expenses. Public perception also plays a role. The word 'nuclear' often conjures images of disasters like Chernobyl or Fukushima, leading to public resistance. Educating the public about the differences between terrestrial nuclear power plants and space-based reactors is essential. Space reactors are typically much smaller, have different designs, and incorporate multiple safety redundancies. Finally, there's the issue of cost. Developing, testing, and deploying NTP systems is an expensive endeavor. While startups and government agencies are investing millions, scaling up to operational levels will require sustained funding. However, proponents argue that the long-term benefits—reduced mission durations, increased payload capacities, and the opening of new mission profiles—justify the initial investment. In summary, while the challenges are non-trivial, they are not insurmountable. Through engineering innovation, rigorous safety protocols, and international cooperation, the obstacles to nuclear thermal propulsion can be addressed.
The Future Impact on Space Exploration
If nuclear thermal propulsion becomes the new norm, it could revolutionize our approach to space travel in ways that are hard to overstate. Faster transit times to Mars not only make crewed missions more feasible but also open up the possibility of more frequent supply runs, the establishment of semi-permanent bases, and even the terraforming of the planet in the distant future. Reduced travel time means astronauts would be less exposed to cosmic radiation and the psychological strains of prolonged isolation. A study by the National Space Biomedical Research Institute in 2022 highlighted that shorter mission durations significantly decrease the risks of space-induced health issues, including bone density loss and muscle atrophy. Beyond Mars, NTP could enable missions to the outer planets that are currently impractical with chemical propulsion. Imagine robotic probes reaching Jupiter or Saturn in a fraction of the current time, allowing for more responsive exploration and data collection. This could accelerate our understanding of the solar system and potentially uncover resources or even life forms that we can't yet imagine. Economically, the adoption of NTP could stimulate new industries, from mining asteroids for rare minerals to space tourism. According to a market analysis by SpaceWorks Enterprises, the space economy could surpass $1 trillion by 2040, with propulsion technologies like NTP playing a pivotal role. Furthermore, military applications cannot be ignored. Faster spacecraft with greater maneuverability could have strategic advantages, prompting interest from defense agencies. This dual-use nature of NTP technology underscores the importance of establishing international norms and agreements to ensure space remains a domain for peaceful exploration. On the flip side, the successful implementation of NTP could also accelerate space debris accumulation, as increased launch rates and more complex missions raise the risk of collisions. This adds urgency to the development of space traffic management and debris mitigation strategies. In essence, nuclear thermal propulsion has the potential to be a catalyst for a new era of space exploration, one where the solar system becomes a playground for scientific discovery, economic development, and perhaps even habitation. The key will be balancing the technological possibilities with the ethical, environmental, and safety considerations that come with wielding such powerful technology.
Conclusion
Nuclear thermal propulsion isn't just a theoretical concept gathering dust in academic journals; it's edging closer to reality, thanks to technological advancements and the relentless pursuit by innovative startups and established agencies alike. It promises to make space travel faster and more efficient, bridging the vast distances of our solar system in ways previously confined to the realm of science fiction. But with great power comes great responsibility (thanks, Uncle Ben). As we stand on the cusp of this new frontier, we must carefully consider the risks and challenges that accompany the rewards. Regulatory frameworks, safety protocols, and public engagement will be as crucial as the engineering feats themselves. So, is NTP the key to deep space exploration, or just another sci-fi fantasy that will fade like so many before it? Only time will tell, but one thing's for sure—it’s an exciting time to keep your eyes on the stars and perhaps start updating your interplanetary travel bucket list. What do you think? Is humanity ready to embrace nuclear propulsion for space travel, or are we venturing into uncharted and unsafe territory? Share your thoughts and let's fuel the conversation!